Advanced Topics in Inorganic Chemistry: Challenging Concepts for Assignments
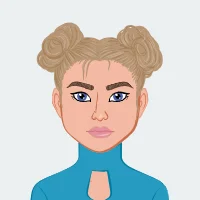
In the vast universe of chemical sciences, inorganic chemistry stands resolute as a foundational pillar, unveiling the enigmatic nature of elements and their compounds. As one traverses the landscape of this discipline, a captivating journey unfolds, revealing intricate details and challenging concepts that define its academic realm. Embracing these complexities not only illuminates the subject matter but also fosters a profound understanding of the fundamental building blocks of matter. If you need assistance with your Inorganic Chemistry assignment, delving into the captivating journey of inorganic chemistry can provide valuable insights and enhance your understanding of the foundational principles underlying chemical compounds and their properties.
At its core, inorganic chemistry delves into the properties, structures, and behaviors of inorganic compounds, encompassing a myriad of elements beyond carbon. It explores the diverse characteristics of metals, non-metals, and metalloids, elucidating their interactions, reactions, and applications. From the electronegativity of atoms to the coordination geometries of complex compounds, every facet beckons exploration, inviting curious minds into its compelling domain.
One of the defining features of inorganic chemistry lies in its penchant for presenting intricate and challenging concepts. Academic assignments within this field often serve as crucibles, testing the mettle of students and researchers alike. Whether deciphering the complexities of coordination compounds or unraveling the mysteries of transition metal chemistry, these tasks demand not just rote memorization but a deep-seated comprehension that transcends surface-level understanding.
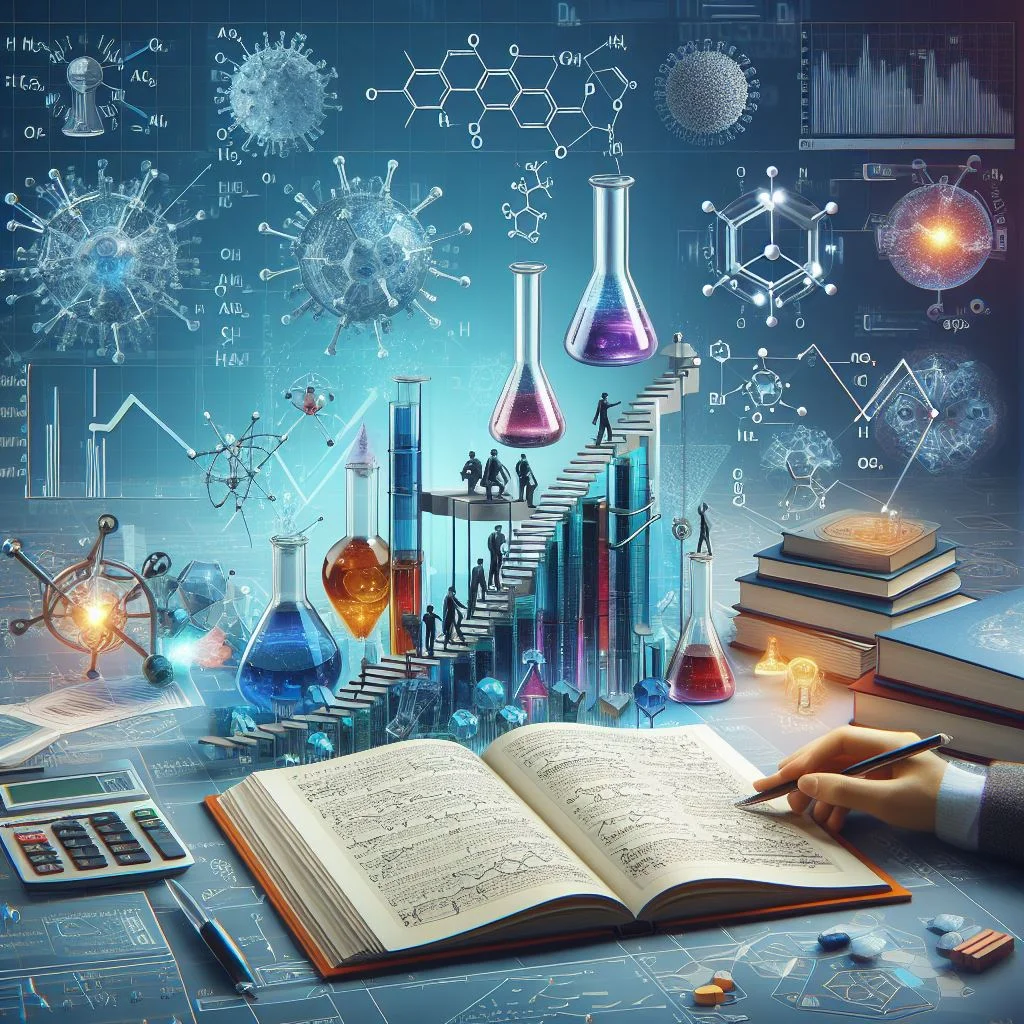
Embracing these challenges is akin to navigating uncharted territory, where each obstacle encountered becomes a stepping stone toward enlightenment. The process of grappling with these complexities instills problem-solving skills, critical thinking, and resilience. It transforms mere students into passionate seekers of knowledge, fostering an appreciation for the depth and breadth of inorganic chemistry.
The journey through inorganic chemistry assignments unveils a tapestry of nuanced facets. From the geometric isomerism in coordination compounds to the diverse applications of organometallic chemistry, each topic paints a vibrant picture of the discipline's diversity. It is a world where the properties of elements shape industries, where catalysts revolutionize chemical processes, and where materials science finds its bedrock.
Moreover, inorganic chemistry extends its reach far beyond the confines of laboratories and textbooks. Its applications reverberate in various sectors, influencing fields as diverse as medicine, agriculture, environmental science, and technology. From the development of novel catalysts for sustainable energy to the utilization of metal complexes in medicinal therapies, its impact resonates across disciplines, shaping the world we inhabit.
In the pursuit of understanding inorganic chemistry, one embarks on an intellectual odyssey, traversing landscapes both familiar and uncharted. It is a journey that transcends the confines of classrooms and laboratories, beckoning individuals to explore the mysteries and complexities that underpin the very fabric of matter.
Unveiling the Complexities of Coordination Chemistry
Coordination chemistry lies at the heart of inorganic chemistry, delving into the intricate interactions between metal ions and ligands within complexes. These compounds are pivotal in comprehending a diverse spectrum of chemical reactions and biological functions. At its core, coordination chemistry scrutinizes the structure, properties, and reactivity of these complexes, unraveling their profound implications in various fields.
Metal ions, acting as the central hub, exhibit diverse coordination numbers and geometries, dictating the arrangement of surrounding ligands. These ligands, with their unique chemical properties, form intricate bonds with the metal center, influencing the stability and behavior of the resulting complex. Understanding these interactions unveils a myriad of applications, from catalysis in industrial processes to the design of targeted pharmaceuticals in medicine.
The study of coordination compounds extends beyond laboratories. It elucidates biological processes like enzymatic reactions and metal transport in living organisms. Moreover, it fuels innovations in materials science, contributing to the development of advanced materials with tailored properties for electronics, optics, and nanotechnology.
In academic assignments, students explore the synthesis, characterization, and applications of coordination compounds, gaining insights into the fundamental principles underpinning this indispensable branch of chemistry.
Ligand Field Theory
The theory concerning electronic structure and bonding in transition metal complexes is pivotal in unraveling the intricate nature of these compounds. Transition metals exhibit diverse properties due to their ability to form complexes with various ligands, influencing their electronic configuration. Understanding the impact of ligands on the splitting of d-orbitals is fundamental. When transition metals combine with ligands, such as ammonia or water, the d-orbitals split in energy, creating different energy levels. This phenomenon, known as crystal field splitting, determines the arrangement of electrons within these orbitals.
This comprehension holds immense significance as it directly correlates to the properties and reactivity of transition metal complexes. For instance, the number of unpaired electrons resulting from the splitting directly impacts the magnetic properties and color observed in certain complexes. Additionally, it plays a pivotal role in dictating the reactivity of these compounds in catalysis or biological processes.
The ability to predict and manipulate these properties empowers scientists in various fields, from materials science to medicinal chemistry. It underpins the design of catalysts, materials with tailored magnetic properties, and drugs targeting specific biological pathways. Thus, the understanding of ligand influence on d-orbital splitting stands as a cornerstone in advancing diverse scientific applications.
Isomerism in Coordination Compounds
Isomerism within coordination complexes presents an intricate realm demanding students' scrutiny. It encompasses two fundamental facets: structural isomerism and stereoisomerism, each a labyrinth of distinct atomic arrangements and consequential properties.
Structural isomerism pertains to compounds sharing a molecular formula but divergent structural layouts. This challenges learners to discern the diverse bonding sequences and spatial orientations of constituent atoms. Such variations induce contrasting chemical behaviors and physical attributes, elucidating the nuanced impacts of structural diversity on complex properties.
Stereoisomerism, on the other hand, explores compounds with identical atomic connectivity but differing spatial orientations in three-dimensional space. The intricate dance of atoms in stereoisomers demands comprehension of their chiral or geometric arrangements, impacting properties like optical activity and reactivity. Understanding these spatial intricacies elucidates the diverse functionalities emerging from identical molecular frameworks.
Students navigating this enigmatic landscape grapple with discerning these atomic choreographies and their implications. The ability to differentiate between these arrangements empowers a comprehensive grasp of how subtle alterations in spatial configurations sculpt the multifaceted behaviors and properties exhibited by coordination complexes, thereby enriching their comprehension of chemical structures at a fundamental level.
Reaction Mechanisms in Transition Metal Complexes
Navigating the intricate world of transition metals within the realm of chemistry presents students with a stimulating yet challenging landscape. Assignments in this domain often task students with unraveling complex reaction pathways involving these elements, which demand a keen understanding of various critical concepts such as oxidation states, ligand substitution, and catalytic cycles.
Transition metals stand distinguished for their multifaceted characteristics, boasting diverse oxidation states, intricate coordination geometries, and remarkable catalytic abilities. Mastery of these properties not only poses significant challenges but also rewards with a profound comprehension of these elements' behaviors and functions within chemical systems.
One of the core focuses of these assignments is delving into the redox chemistry of transition metals. This exploration serves to unveil the pivotal role these metals play in electron transfer processes. Redox reactions involving transition metals often constitute the crux of these assignments, prompting students to balance complex equations, predict oxidation states, and comprehend the intricate dance of electrons within these reactions. The ability to decipher and manipulate these redox processes is fundamental to grasping the essence of transition metals' involvement in chemical reactions and their broader significance in various applications, from industrial processes to biological systems.
Deciphering reaction pathways involving transition metals requires a firm grasp of oxidation states, a fundamental concept pivotal in understanding the electron distribution within these elements. Ligand substitution, another intricate facet, explores the dynamics of ligands binding and releasing from transition metal ions, impacting the overall reactivity and behavior of these compounds. Furthermore, understanding catalytic cycles unveils the mechanisms through which these metals accelerate reactions without being consumed, shedding light on the efficiency and selectivity of these catalysts.
The complexity of these assignments not only challenges students' problem-solving skills but also fosters a deeper appreciation for the elegance and sophistication of transition metals. The ability to navigate through these complexities fosters not just academic growth but also cultivates a mindset attuned to exploring the subtleties and intricacies of chemical reactions.
Crystal Field Theory
In the realm of inorganic chemistry, the intricate dance between ligands and metal ions within transition metal complexes unveils a fascinating interplay of electronic configurations. This interplay, elucidated by Crystal Field Theory, not only enriches our comprehension of molecular interactions but also serves as a cornerstone for predicting and understanding distinctive properties such as color and magnetic behavior.
Transition metal complexes find themselves at the heart of numerous applications spanning catalysis, medicine, and materials science. Their unique structures and versatile reactivities empower them to act as catalysts in fundamental chemical transformations, catalyzing reactions that might otherwise proceed sluggishly or remain inert. In medicine, these complexes demonstrate promising potential as therapeutic agents or imaging agents due to their tailored properties and specific interactions with biological systems. Moreover, their integration into materials science applications leads to the creation of novel materials with tailored properties, contributing to advancements in various technologies.
Understanding these complexes in depth requires an exploration of the intricate structure-function relationships that govern their behavior. Inorganic chemistry assignments often delve into unraveling the mysteries of molecular structures, demanding a firm grasp of three-dimensional arrangements of atoms. Spectroscopic techniques serve as indispensable tools in this pursuit, offering insights into these complex structures. Among these techniques, X-ray Crystallography stands as a pivotal method, enabling the determination of precise atomic arrangements in crystals. This profound understanding of molecular architecture aids in predicting properties and behaviors, paving the way for innovative applications across scientific disciplines.
X-ray Crystallography, with its ability to unveil the hidden world of molecular arrangements, plays a pivotal role. By subjecting crystals to X-ray diffraction, scientists unravel the precise spatial arrangements of atoms within molecules. This not only sheds light on their geometric arrangements but also provides a roadmap for understanding their behavior and interactions with their surroundings.
Inorganic chemistry assignments often task students with navigating these realms, diving deep into the realms of ligand-metal interactions, crystal field theory, and the practical applications of transition metal complexes. It's an exploration that not only unlocks the mysteries of molecular structures but also unveils a spectrum of possibilities for transformative scientific discoveries across various fields. As students delve into these topics, they unravel the intricate tapestry of inorganic chemistry, poised at the intersection of theory, experimentation, and real-world applications.
Spectroscopic Techniques
Assignments within the realm of chemistry often entail a multifaceted approach, intricately weaving together data interpretation from diverse spectroscopic techniques like UV-Vis, IR, NMR, and Mass Spectrometry. These methods serve as invaluable tools, each offering a unique lens through which molecular structures can be deduced and the nuanced tapestry of chemical properties can be unraveled.
The utilization of UV-Vis spectroscopy unveils insights into electronic transitions within molecules, shedding light on their bonding patterns and the presence of conjugated systems. Meanwhile, Infrared (IR) spectroscopy probes molecular vibrations, allowing for the identification of functional groups and structural elucidation. Nuclear Magnetic Resonance (NMR) spectroscopy delves into the magnetic properties of atomic nuclei, providing a treasure trove of information about molecular connectivity and environments. Furthermore, Mass Spectrometry unravels the molecular mass and fragmentation patterns, aiding in the determination of elemental composition and structural validation.
However, the comprehension of molecular symmetry emerges as an equally pivotal facet in these assignments. Understanding symmetry elements and operations becomes the compass guiding predictions about molecular properties and behavior under spectroscopic scrutiny. The symmetry operations elucidate how molecules transform under rotations, reflections, and inversions, unveiling critical insights into their spectroscopic behavior.
The amalgamation of these methodologies forms the backbone of assignments geared towards structure determination. Analyzing spectral data through the lens of UV-Vis, IR, NMR, and Mass Spectrometry allows chemists to piece together molecular puzzles. Concurrently, the adept utilization of symmetry principles assists in predicting and rationalizing spectroscopic outcomes, propelling the accurate determination of molecular structures.
Conclusion
Inorganic chemistry, a realm of scientific exploration delving into the properties, behavior, and interactions of elements and their compounds, offers a vast terrain of complex and intriguing concepts. Within this discipline lie multifaceted dimensions awaiting exploration, challenging aspiring chemists and seasoned enthusiasts alike. Embracing the labyrinth of intricacies within inorganic chemistry assignments not only presents formidable challenges but also serves as a portal to a profound comprehension of the subject matter.
At the heart of inorganic chemistry lies a tapestry woven from diverse elements and compounds, each with its own unique characteristics and behaviors. Coordination chemistry, a captivating realm within this discipline, unveils the intricate dance of metal ions and ligands, revealing the complexities of molecular architecture and bonding. Transition metals, with their versatile oxidation states and multifunctional nature, beckon scientists to decipher their nuances, enabling advancements in catalysis, materials science, and beyond.
Molecular structures stand as the fundamental building blocks that define the behavior and properties of compounds. Inorganic chemistry navigates through an array of molecular architectures, elucidating the structural intricacies that govern reactivity, stability, and functionality. Understanding these structures becomes a pivotal cornerstone in unraveling the mysteries of inorganic compounds.
Spectroscopy, a powerful analytical tool, serves as a guiding light in the exploration of inorganic chemistry. It allows us to peer into the molecular world, unraveling the secrets held within atomic and molecular spectra. Spectroscopic techniques, ranging from UV-Vis to NMR and beyond, empower chemists to decode molecular fingerprints, unravel electronic configurations, and comprehend the behavior of compounds under varying conditions.
The path through inorganic chemistry assignments, while laden with challenges, unveils a journey ripe with opportunities for enlightenment. For students embarking on this expedition, these challenges act as stepping stones toward a deeper understanding of the subject matter. Each hurdle overcome signifies a conquest in deciphering the complexities of inorganic compounds and their behaviors.
Simultaneously, for the curious minds of chemistry enthusiasts, diving into these advanced topics serves as an odyssey through uncharted territories. Exploring the depths of coordination chemistry, unraveling the mysteries of transition metals, deciphering molecular structures, and decoding spectral signatures become pursuits that enrich one's appreciation for the elegance and intricacy of inorganic chemistry.
Embracing these advanced topics is not merely an academic exercise but a transformative journey. It fosters a holistic comprehension of the intricate relationships between structure and reactivity, unlocking doors to innovative applications and discoveries. Mastery in inorganic chemistry is not solely about overcoming challenges; it's about assimilating the diverse facets of this discipline into a comprehensive mosaic of knowledge and understanding.
In conclusion, the realm of inorganic chemistry beckons with its complexities, offering a tapestry of challenges waiting to be embraced. Whether as a student navigating assignments or an enthusiast delving into the depths of this captivating field, the journey through these advanced topics promises not just knowledge but a profound appreciation for the mysteries and marvels of inorganic chemistry.