Molecular Biology Techniques: Experimental Methods for Biochemistry Assignments
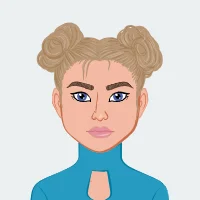
Molecular biology techniques are the backbone of biochemistry, providing the essential tools to unravel the intricate details of cellular processes and decode genetic information. This comprehensive guide aims to shed light on the complexities of various experimental methods crucial for Biochemistry assignments. Whether you are a student embarking on the journey into the captivating realm of molecular biology or a seasoned researcher in need of a refresher, this exploration promises valuable insights into techniques like DNA sequencing, Polymerase Chain Reaction (PCR), Western blotting, and gel electrophoresis. Understanding molecular biology techniques is essential for mastering the experimental methods used in biochemistry research and analysis, ensuring success in your academic pursuits.
At the heart of molecular biology lies the quest to understand the fundamental building blocks of life. DNA sequencing stands out as a pivotal technique, allowing scientists to decipher the genetic code with unparalleled precision. By determining the order of nucleotides in a DNA molecule, researchers gain a roadmap to unravel the mysteries of genes, paving the way for advancements in genetics, genomics, and personalized medicine.
Polymerase Chain Reaction, commonly known as PCR, is another indispensable tool in the molecular biologist's arsenal. This technique amplifies specific DNA sequences, enabling the generation of millions of copies from a minute starting material. Widely used in various applications, from genetic fingerprinting to studying gene expression, PCR has revolutionized the speed and efficiency of DNA analysis.
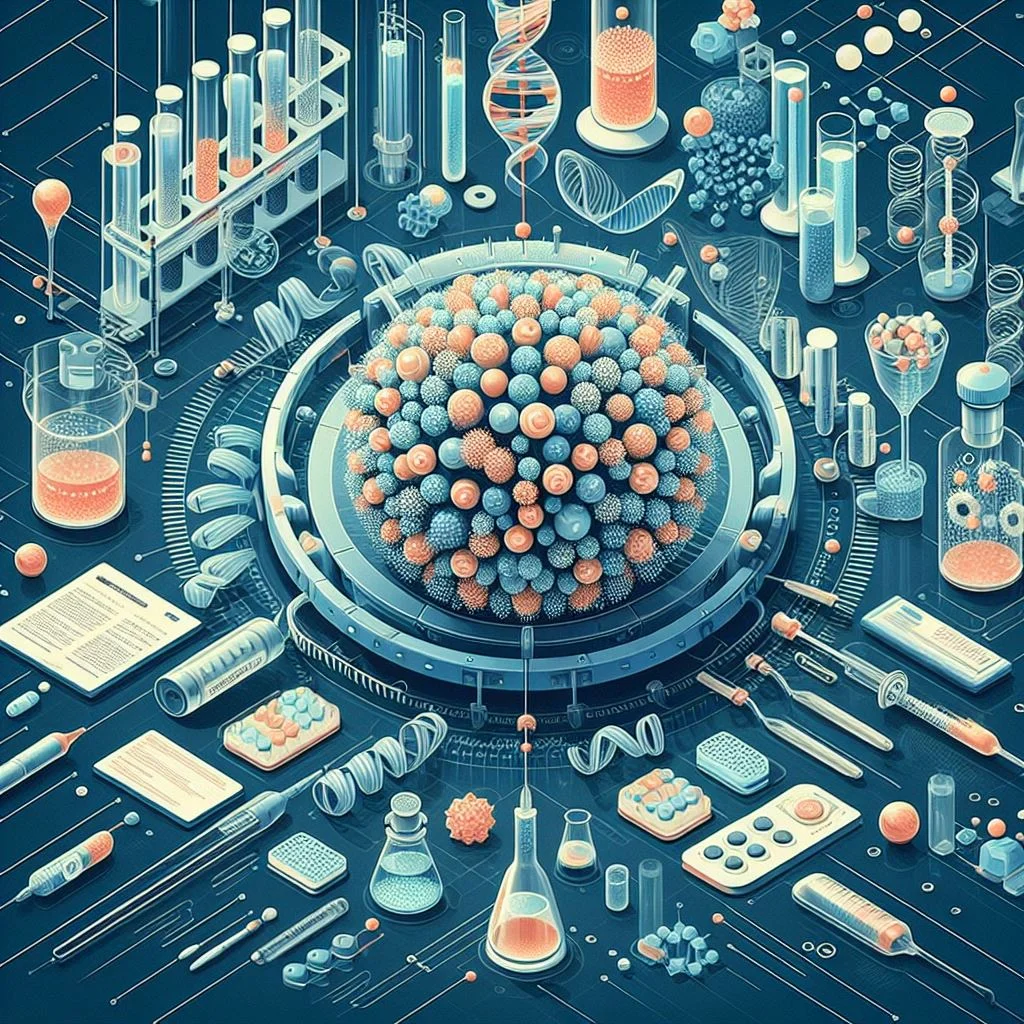
Moving beyond DNA manipulation, Western blotting is a technique employed to detect specific proteins within a sample. This method involves separating proteins through gel electrophoresis, transferring them to a membrane, and then probing with antibodies to visualize the target proteins. Western blotting plays a crucial role in understanding protein expression levels, post-translational modifications, and interactions, contributing significantly to the fields of molecular biology and biochemistry.
Gel electrophoresis, a cornerstone technique, allows the separation of macromolecules such as DNA, RNA, or proteins based on size and charge. By subjecting the molecules to an electric field, they migrate through a gel matrix, creating distinct bands that can be visualized and analyzed. This method is essential for tasks ranging from DNA fingerprinting to the analysis of protein purity.
DNA Sequencing: Decoding the Genetic Blueprint
DNA sequencing is a pivotal molecular biology technique that plays a crucial role in unraveling the mysteries encoded within the genetic material of living organisms. At its core, DNA sequencing involves determining the precise order of nucleotides in a given DNA molecule. This process provides a comprehensive understanding of the genetic code, enabling researchers to delve into the intricacies of life at the molecular level.
One of the foundational methods in DNA sequencing is Sanger sequencing, named after its inventor, Frederick Sanger. This technique relies on the synthesis of DNA chains and the incorporation of chain-terminating dideoxynucleotides during replication. The resulting fragments are then separated by size, revealing the sequence of nucleotides. Sanger sequencing has been instrumental in decoding genomes, including the landmark Human Genome Project.
In recent years, next-generation sequencing (NGS) has revolutionized the field, offering high-throughput and parallel sequencing capabilities. NGS techniques, such as Illumina sequencing and Ion Torrent sequencing, have significantly reduced the time and cost associated with DNA sequencing. This has paved the way for unprecedented advancements in genomics, transcriptomics, and epigenomics research.
The applications of DNA sequencing in biochemistry assignments are diverse and impactful. Genetic disease diagnosis stands out as a critical application, where identifying genetic mutations and variations helps in understanding the underlying causes of hereditary disorders. Evolutionary studies benefit from DNA sequencing by unraveling the genetic changes that have shaped the diversity of life over millennia. Functional genomics, another key area, relies on sequencing data to elucidate the roles and interactions of genes in biological processes.
Polymerase Chain Reaction (PCR): Amplifying DNA for Analysis
Polymerase Chain Reaction (PCR) stands at the forefront of molecular biology as a revolutionary technique, providing researchers with the ability to amplify specific DNA sequences with unparalleled precision. This groundbreaking method has become a cornerstone in various scientific applications, unraveling the mysteries encoded within the DNA strands.
At its core, PCR relies on a series of thermal cycling processes to exponentially replicate target DNA. Three main components drive this amplification: DNA template, primers, and DNA polymerase. The template, typically a segment of DNA containing the target sequence, serves as the foundation. Primers, short single-stranded sequences that complement the target DNA, initiate the replication process. DNA polymerase, a specialized enzyme, extends the primers, synthesizing new DNA strands. The thermal cycling involves repeated cycles of denaturation, annealing, and extension, ensuring the selective amplification of the desired DNA sequence.
Understanding these fundamental principles is crucial for harnessing the power of PCR in various applications, from basic research to medical diagnostics.
The versatility of PCR extends beyond its basic form, giving rise to variations tailored to specific research needs. Quantitative PCR (qPCR) provides a quantitative measure of the DNA present in a sample, offering insights into gene expression levels. Reverse Transcription PCR (RT-PCR) is employed to analyze RNA by converting it into complementary DNA (cDNA) before amplification. These variations have become indispensable tools in biochemistry research, contributing to the study of gene expression, disease diagnostics, and molecular cloning.
The role of PCR in gene expression analysis is pivotal, allowing researchers to decipher how genes are activated or silenced under different conditions. In molecular diagnostics, PCR enables the detection of pathogens and genetic mutations, offering a rapid and sensitive approach for disease diagnosis. Additionally, PCR plays a crucial role in cloning applications, facilitating the creation of multiple copies of a gene for further study or manipulation.
Western Blotting: Detecting Proteins with Precision
Western blotting is a fundamental technique widely employed in molecular biology to identify and quantify specific proteins within complex biological samples. At its core, Western blotting involves several key principles, including gel electrophoresis, protein transfer, and antibody detection.
Gel electrophoresis is the initial step where proteins are separated based on their size through a porous gel matrix. This separation allows for the identification of individual proteins within a mixture. Subsequently, the separated proteins are transferred from the gel onto a membrane, usually made of nitrocellulose or polyvinylidene difluoride (PVDF). This transfer process is crucial for further analysis as it facilitates the probing of specific proteins.
The heart of Western blotting lies in the detection of target proteins using antibodies. These antibodies are designed to bind specifically to the protein of interest. After the protein transfer, the membrane is incubated with these antibodies, allowing for the formation of antibody-protein complexes. The visualization of these complexes is achieved through various methods, such as chemiluminescence or fluorescent tags, enabling the identification and quantification of the targeted proteins.
Beyond its foundational principles, Western blotting finds extensive applications in biochemical research. Researchers use this technique for diverse purposes, including the analysis of protein expression levels, investigating post-translational modifications, and diagnosing clinical conditions. The ability to visualize and quantify specific proteins makes Western blotting an invaluable tool in understanding cellular processes and identifying biomarkers associated with diseases.
Despite its power, Western blotting can be prone to challenges. Common issues include inadequate protein transfer, non-specific binding of antibodies, and variability in experimental conditions. Troubleshooting these problems is crucial for obtaining reliable and reproducible results.
Gel Electrophoresis: Separating Molecules with Precision
Gel electrophoresis stands as a foundational technique in molecular biology, facilitating the separation of macromolecules such as nucleic acids and proteins based on their size and charge. This method employs specialized gels, commonly made of agarose or polyacrylamide, which act as a matrix through which molecules can migrate when subjected to an electric field. The separation is accomplished as molecules navigate the gel, with smaller and more highly charged particles moving faster than larger, less charged ones. This simple yet powerful principle forms the bedrock of gel electrophoresis, allowing scientists to dissect complex mixtures into distinct components for further analysis.
Beyond the fundamentals lie advanced gel electrophoresis techniques that elevate the precision and resolution of molecular separation. Pulsed-Field Gel Electrophoresis (PFGE) introduces a nuanced approach by employing alternating electric field pulses in multiple directions. This is particularly valuable when dealing with large DNA fragments, enhancing the resolution of separation in biochemistry experiments.
Two-Dimensional Gel Electrophoresis (2D-GE) takes the technique to a higher dimension, incorporating a second property for separation. Typically, molecules are separated based on isoelectric point in one dimension and molecular weight in the other. This dual-axis separation provides a more detailed view of complex mixtures, allowing researchers to distinguish closely related molecules that might appear as a single band in traditional gel electrophoresis.
Biochemical Analysis: Integrating Techniques for Comprehensive Insights
The integration of multiple molecular biology techniques stands as a cornerstone in modern biochemical analysis, offering a holistic approach to unravel the complexities of biological systems. At the forefront of this integration are techniques such as DNA sequencing, Polymerase Chain Reaction (PCR), Western blotting, and gel electrophoresis. These methodologies, when strategically combined, yield a more profound understanding of intricate biological questions. The significance lies not only in the individual strengths of each technique but in their synergistic application.
DNA sequencing unveils the genetic blueprint, while PCR amplifies specific DNA sequences, facilitating their detailed analysis. Western blotting enables the identification and quantification of proteins, and gel electrophoresis separates molecules based on size. When seamlessly integrated, these techniques complement one another, providing a comprehensive view of the molecular landscape. Case studies play a pivotal role in illustrating the power of integration, showcasing scenarios where a collective approach unravels biological mysteries that might remain obscured when relying on a single technique.
In parallel, the molecular biology landscape is continually evolving with the advent of groundbreaking technologies. Staying abreast of these innovations is imperative for researchers aiming to push the boundaries of biochemistry. Among the forefront technologies are CRISPR-Cas9 genome editing, enabling precise manipulation of genetic material. Single-cell sequencing delves into the intricacies of individual cells, unraveling heterogeneity within populations. Mass spectrometry-based proteomics allows for the identification and quantification of proteins, revolutionizing our understanding of cellular function.
These emerging technologies are reshaping the research paradigm, offering unprecedented opportunities for exploration and discovery. CRISPR-Cas9, in particular, has revolutionized genetic engineering, providing a versatile tool for modifying genes with high precision. Single-cell sequencing has unveiled a new dimension in understanding cellular diversity, allowing researchers to study individual cells within complex tissues. Mass spectrometry-based proteomics, on the other hand, has transformed our ability to analyze the proteome, shedding light on the dynamic nature of cellular processes.
Conclusion
As we embark on the concluding leg of our exploration into the intricate realm of molecular biology techniques, it becomes undeniably clear that these experimental methods serve as the backbone of biochemistry assignments. In the intricate dance of life's molecular processes, from the blueprint encoded in our genes to the nuanced detection of proteins, each technique acts as a sentinel, contributing to our profound understanding of the complex biochemical choreography that orchestrates life itself.
One of the primary facets of molecular biology involves the unravelling of the genetic blueprint encoded in our DNA. Decoding this intricate script is akin to deciphering the language of life. Through techniques such as DNA sequencing, researchers can meticulously read and interpret the sequence of nucleotides, unlocking the secrets held within our genes. This foundational step not only informs us about the genes themselves but also provides critical insights into genetic variations, mutations, and the potential implications for health and disease.
The amplification of DNA is another cornerstone in the molecular biologist's toolkit. Techniques like polymerase chain reaction (PCR) allow for the targeted and exponential replication of specific DNA segments. This process is indispensable in various applications, including genetic research, diagnostics, and forensic analysis. Amplifying DNA enables researchers to obtain sufficient material for detailed analysis, making it a pivotal step in studying genes, identifying pathogens, and understanding the genetic basis of diverse biological phenomena.
In the delicate orchestration of cellular functions, proteins take center stage. Detecting and analyzing proteins with precision is a paramount aspect of molecular biology, shedding light on the intricacies of cellular processes. Techniques such as western blotting, enzyme-linked immunosorbent assay (ELISA), and mass spectrometry enable scientists to identify, quantify, and characterize proteins. These methods play a vital role in unraveling the mysteries of cell signaling, metabolic pathways, and the dynamic interplay of biomolecules within living organisms.
Armed with the insights garnered from these molecular biology techniques, students and researchers alike are equipped to navigate the complex landscape of biochemistry. The comprehensive guide provided serves as a beacon, illuminating the path to excellence in biochemistry endeavors. Whether delving into the intricacies of genetic disorders, exploring novel drug targets, or deciphering the signaling cascades within cells, a solid foundation in molecular biology techniques is indispensable.
In conclusion, the journey through molecular biology techniques unveils their indispensable role in biochemistry assignments. These methods, from decoding the genetic script to amplifying DNA and scrutinizing proteins with precision, collectively contribute to our comprehension of the nuanced biochemical processes governing life. As we traverse the molecular landscape armed with this comprehensive guide, we are not merely observers but active participants in unraveling the profound mysteries of the molecular world.