Bridging Theory with Practice through Molecular Modeling
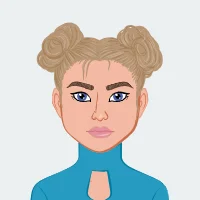
Physical Chemistry stands at the intersection of physics and chemistry, delving into the foundational principles governing the behavior of chemical systems. It is an expansive discipline that scrutinizes molecular structures, interactions, and dynamics, unraveling the intricacies of matter at its fundamental level. Amidst this exploration, one of its pivotal tools emerges: Molecular Modeling. If you need assistance with your Physical Chemistry assignment, understanding the role of molecular modeling in analyzing chemical systems can provide valuable insights for your assignment.
The evolution of Physical Chemistry has been significantly influenced by the advancement of computational techniques. These technological strides have revolutionized the study of molecular phenomena, ushering in an era where theoretical concepts can be vividly simulated and observed. The marriage between computation and chemistry has transformed the way scientists comprehend and analyze molecular structures and behaviors. With the aid of high-powered computers and sophisticated algorithms, researchers can simulate molecular interactions, predict properties, and elucidate complex chemical processes that would otherwise be daunting to investigate solely through experimental means.
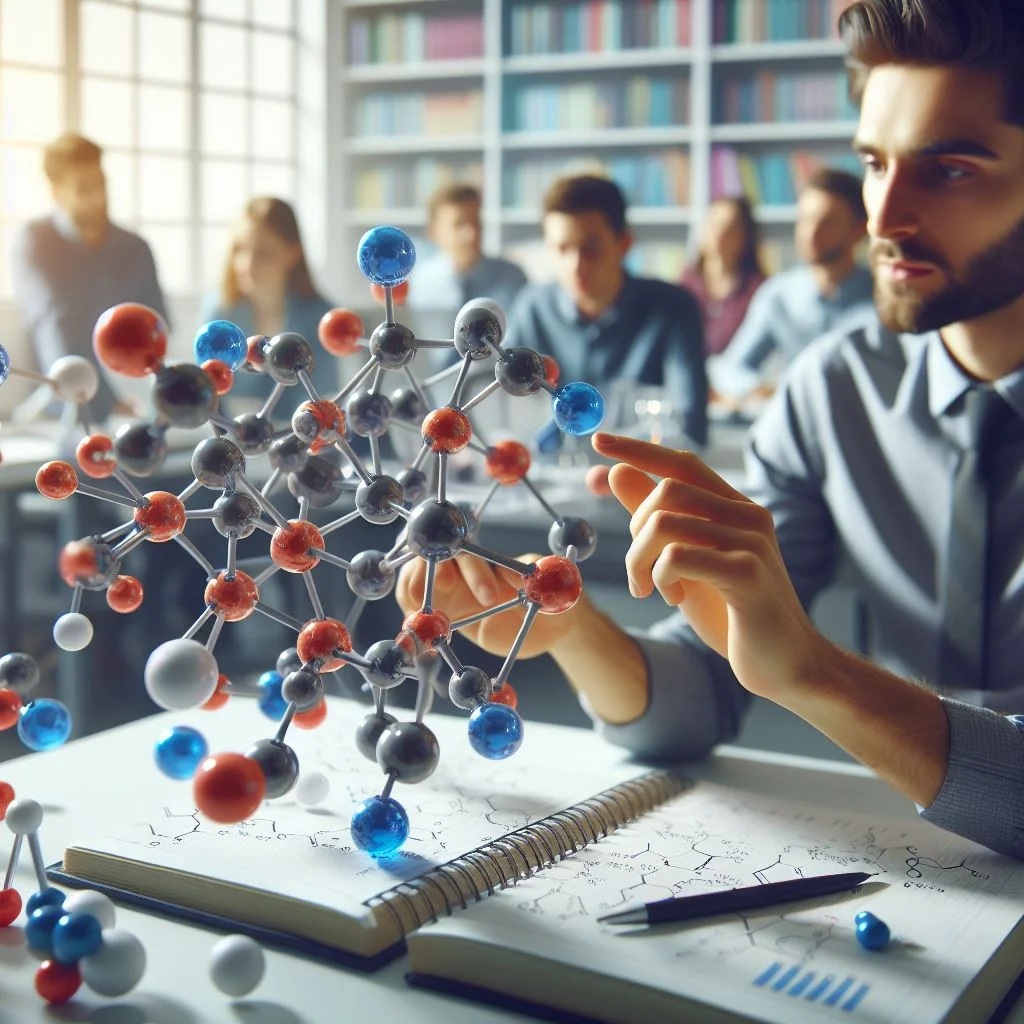
Molecular modeling, a cornerstone of Physical Chemistry, serves as a potent instrument that offers a portal into the microscopic realm of molecules. It enables chemists and researchers to navigate this unseen world, allowing them to visualize and manipulate molecules in ways that were once unimaginable. This field encompasses various methods, such as molecular mechanics, quantum chemistry, and molecular dynamics, each tailored to probe specific aspects of molecular behavior.
At the heart of molecular modeling lies its ability to aid academic pursuits within the realm of Physical Chemistry assignments. It provides a virtual laboratory where students can explore and comprehend theoretical concepts in a tangible manner. Through computational simulations, students can gain insights into molecular structures, predict molecular properties, and understand the dynamics of chemical reactions. This not only enhances their comprehension but also fosters a deeper appreciation for the underlying principles governing chemical systems.
One of the primary advantages of molecular modeling in academic assignments is its capacity to bridge the gap between theory and experimentation. It allows students to apply theoretical knowledge to practical scenarios, enabling them to test hypotheses and explore diverse chemical phenomena. Moreover, molecular modeling provides a platform for students to engage in hands-on learning, encouraging critical thinking and problem-solving skills essential for future scientific endeavors.
The significance of molecular modeling in aiding assignments within Physical Chemistry is multifaceted. It serves as a catalyst for innovation, enabling students to explore cutting-edge technologies and methodologies employed in modern research. Additionally, it cultivates a deeper understanding of the intricate world of molecules, laying the groundwork for future scientific advancements and discoveries.
Understanding Molecular Modeling in Physical Chemistry
Molecular modeling is an intricate process leveraging computational tools to mimic and analyze the behavior, structure, and interactions of molecules. At its essence, it operates through an amalgamation of theoretical frameworks and mathematical algorithms that replicate the dynamic nature of atoms and molecules in varying environments.
By utilizing sophisticated software and algorithms, molecular modeling elucidates complex molecular structures and their properties, providing insights into their behavior under different conditions. This computational approach aids in predicting molecular behaviors, such as reaction pathways, stability, and binding affinities, which are invaluable in drug design, material science, and biochemical research.
Through molecular dynamics simulations, these models mimic the movements and interactions of atoms over time, offering a dynamic representation of molecular behavior. Additionally, quantum mechanics-based calculations delve into electronic structures and energies, enabling a deeper understanding of chemical phenomena at a fundamental level.
Ultimately, molecular modeling serves as a pivotal tool, augmenting experimental techniques and accelerating scientific discovery. Its ability to predict and comprehend molecular behavior empowers researchers across diverse fields, fostering advancements in pharmaceuticals, materials science, and various branches of chemistry.
Types of Molecular Modeling Techniques
Simulations play a pivotal role in unraveling the intricate behaviors of molecules and materials, offering a window into their fundamental properties at a microscopic level. Among these simulations, Quantum Mechanics (QM), Molecular Dynamics (MD), and Monte Carlo simulations stand as essential tools in the arsenal of computational chemistry and physics.
Quantum Mechanics simulations serve as a virtual microscope, probing the electronic structure of molecules. By delving into the realm of quantum states, these simulations elucidate the energy levels that electrons occupy within atoms and molecules. They unravel the complex dance of electrons, detailing how they form bonds between atoms and the underlying mechanisms orchestrating chemical reactions. This deep dive into quantum-level phenomena provides invaluable insights, aiding in the design of novel materials and understanding reaction mechanisms with remarkable precision.
In contrast, Molecular Dynamics simulations take a dynamic stance, observing the movements and interactions of atoms and molecules over time. By tracing the trajectories of particles, these simulations capture the fluid, ever-changing nature of molecular systems. They unveil not just static structures, but the dynamic changes occurring within a system. This dynamic perspective is invaluable in studying thermodynamic properties, phase transitions, and how materials respond to various conditions.
Meanwhile, Monte Carlo simulations adopt a statistical approach, predicting molecular behavior through probabilistic assessments. By sampling various configurations based on statistical probabilities, these simulations navigate the vast configuration space of molecules. They explore phase transitions, equilibrium states, and the likelihood of certain events occurring within a system. This statistical methodology provides valuable insights, particularly in scenarios where deterministic modeling becomes impractical due to complexity.
Collectively, these simulations serve as computational laboratories, allowing scientists to explore and understand the behaviors of molecules and materials in ways that are often impossible or impractical through traditional experimental approaches. Their synergy empowers researchers to unravel the mysteries of molecular interactions, enabling advancements in fields ranging from material science to drug discovery, fostering innovation and deeper comprehension of the fundamental building blocks of our universe.
Applications in Physical Chemistry Assignments
Molecular modeling stands as a pivotal tool in modern chemistry, revolutionizing the way we comprehend, analyze, and predict molecular behaviors. Its multifaceted applications encompass a spectrum of crucial areas within the realm of chemistry, delineating its profound significance in scientific exploration.
One of its primary roles lies in structure elucidation. Through molecular modeling, scientists decode intricate molecular geometries, unraveling stable conformations, and predicting essential molecular properties. This methodological approach serves as the cornerstone for assignments where understanding the structure of compounds is paramount. By visualizing and manipulating molecular structures in a simulated environment, researchers gain insights into the arrangement of atoms, leading to comprehensive structural elucidation.
Moreover, molecular modeling finds extensive use in studying thermodynamics and kinetics. Simulations facilitate the exploration of reaction pathways, determination of rate constants, and unraveling the intricate tapestry of thermodynamic properties. These investigations provide invaluable insights, enabling a deeper comprehension of chemical kinetics and thermodynamics. Assignments centered around understanding the driving forces behind reactions, their rates, and associated energy changes benefit immensely from these predictive capabilities.
Additionally, property prediction stands as another crucial facet of molecular modeling. By leveraging computational techniques, scientists predict molecular energies, electronic structures, and spectroscopic properties with remarkable accuracy. This predictive prowess aids in assignments focused on unraveling the intricate nuances of chemical behaviors and properties, empowering researchers to anticipate and comprehend the behavior of molecules under diverse conditions.
Collectively, these applications signify the profound impact of molecular modeling across various branches of chemistry. Its ability to decipher complex molecular structures, unveil reaction mechanisms, and predict molecular properties not only enhances our understanding of fundamental chemical principles but also catalyzes advancements in drug design, material science, and various other scientific domains. As technology continues to evolve, the role of molecular modeling in shaping the landscape of chemistry remains indispensable, fostering innovation and driving scientific exploration to unprecedented heights.
The Role of Molecular Modeling in Enhancing Learning and Research
Molecular modeling stands as a transformative bridge in the realm of scientific exploration and education, wielding its power to render abstract theories into vivid, tangible representations. Within this realm, its impact resonates across three pivotal domains.
Firstly, its ability to visually manifest abstract concepts heralds a new era in comprehension. In the intricate world of molecules and compounds, where theories often reside in the realm of the intangible, molecular modeling serves as an artist's brush, painting these elusive concepts into comprehensible and engaging visualizations. Students, often grappling with the complexities of theoretical frameworks, find solace and clarity as these once-elusive ideas take form before their eyes. Such visual aids serve not just as educational tools but as catalysts for curiosity and understanding.
Beyond mere illustration, molecular modeling assumes the mantle of a virtual laboratory, a canvas for experimentation and hypothesis validation. Here, researchers and students alike delve into simulations, a sandbox where theory meets reality. These simulations provide a platform for comparative analysis, enabling the validation of experimental findings or the rigorous testing of hypotheses against empirical data. This iterative process fortifies scientific reasoning, nurturing a generation adept at critically assessing theories and data through a lens refined by empirical verification.
Yet, perhaps its most profound impact lies in its role as an incubator of innovation. In the laboratory of molecular modeling, the shackles of traditional experimental constraints loosen, inviting exploration into the uncharted territories of unconventional ideas and hypotheses. Researchers and students, unencumbered by logistical limitations, can venture forth to test hypotheses that might otherwise remain relegated to the realm of impossibility through traditional experimental methods. It's here, amidst this boundless frontier, where innovation thrives and paradigm-shifting discoveries unfold.
In essence, molecular modeling transcends the boundaries of traditional pedagogy and research methodologies, ushering in an era where the abstract becomes tangible, experimentation transcends physical limitations, and innovation finds fertile ground to flourish. Its impact reverberates not just within the confines of scientific inquiry but also in the nurturing of inquisitive minds, fostering a generation adept at navigating the complexities of the molecular world and beyond.
Tools and Software for Molecular Modeling Assignments
In the realm of molecular modeling, software plays a pivotal role, offering diverse tools catering to various needs. Open-source platforms like Avogadro, GROMACS, and Quantum Espresso stand as pillars of accessibility, providing potent molecular modeling tools at no cost. Their availability empowers educational endeavors, granting students and researchers powerful resources for understanding molecular structures and properties.
On the other hand, commercial software like Gaussian, Schrödinger, and Materials Studio embodies sophistication, delivering comprehensive suites for intricate simulations and analyses. Widely utilized across academia and industries, these platforms offer cutting-edge capabilities, driving innovation in drug discovery, material science, and theoretical chemistry. Their robustness and advanced features make them indispensable in tackling complex molecular modeling challenges, elevating scientific exploration and industrial development.
The symbiotic existence of open-source and commercial software in molecular modeling signifies a dynamic balance. Open-source tools foster accessibility and learning, while commercial software propels intricate research and development. Together, they form a landscape where accessibility and sophistication converge, catalyzing advancements in understanding molecular intricacies and propelling innovation in diverse scientific domains.
Challenges and Future Perspectives
Molecular modeling stands as a cornerstone in scientific inquiry, unveiling the mysteries of molecular behavior and enabling insights into complex systems. Yet, amid its transformative potential, several challenges persist, shaping the trajectory of its evolution and driving the quest for innovation.
One of the paramount hurdles in molecular modeling is the conundrum of computational resource limitations. The intricate nature of molecular systems demands substantial computational power for simulations, especially when navigating complex structures or studying large-scale systems. However, the accessibility and availability of high-powered computing resources often pose barriers, constraining the scope and depth of simulations. This limitation not only impedes progress but also restrains the exploration of intricate molecular phenomena.
An inherent pursuit within molecular modeling is the perpetual quest for enhanced model accuracy. The fidelity of simulations to real-world experimental observations remains a critical pursuit. Continuous advancements are imperative to refine modeling algorithms, ensuring that simulated outcomes align more closely with empirical data. Achieving higher accuracy augments the reliability and applicability of molecular models, fortifying their utility in diverse scientific domains.
Amid these challenges, an avenue of promising innovation emerges through the integration of AI and machine learning techniques. This synergy holds tremendous potential in revolutionizing molecular modeling by augmenting predictive capabilities and expediting simulations. AI's capacity to discern patterns and optimize processes presents an opportunity to streamline the modeling workflow. Integrating machine learning not only facilitates the handling of vast datasets but also empowers models to learn and adapt, potentially revolutionizing the precision and efficiency of molecular simulations.
Efforts to overcome these challenges are vital for the advancement of molecular modeling. Addressing computational limitations requires initiatives to enhance access to powerful computing resources or develop more efficient algorithms that optimize resource utilization. Moreover, a relentless commitment to refining modeling techniques and leveraging AI-driven innovations is pivotal. Collaborative endeavors between computational scientists, chemists, and experts in AI and machine learning will catalyze breakthroughs, enabling a more profound understanding of molecular complexities and expanding the frontiers of scientific exploration.
In the evolving landscape of molecular modeling, the convergence of computational prowess, algorithmic refinement, and AI integration holds the promise of unlocking unprecedented insights into molecular structures and interactions. By navigating and surmounting these challenges, the scientific community paves the way for a new era of discovery, innovation, and transformative applications across various scientific disciplines and industries.
Conclusion:
Molecular modeling, an indispensable tool in physical chemistry, stands as a testament to humanity's quest to decipher the intricate behaviors and structures of molecules that compose our universe. Its significance resonates through the corridors of academia, research laboratories, and technological advancements, revolutionizing the comprehension of molecular phenomena.
At its core, molecular modeling acts as a guiding torch, illuminating the otherwise enigmatic pathways of molecular behavior. Through computational algorithms and simulations, it unlocks the Pandora's box of molecular intricacies, unveiling the three-dimensional dance of atoms and molecules. This unveiling not only aids in understanding but also paves the way for innovation, pushing the boundaries of scientific exploration.
Within the academic sphere, the integration of molecular modeling transforms the educational landscape. Students and researchers are not mere spectators but active participants, wielding computational tools to navigate the abstract concepts of physical chemistry. This integration transcends traditional learning, fostering critical thinking and problem-solving skills. It's a journey where textbooks come alive, where theoretical concepts find tangible expressions in virtual molecular structures, enriching the educational experience.
Yet, beyond academia, this amalgamation of theory and computation propels the realms of research and discovery. As technology continues its inexorable march forward, molecular modeling becomes the vanguard of innovation. It complements experimental observations, guiding scientists toward uncharted territories, predicting chemical behaviors, and accelerating the development of new materials, drugs, and technologies. It is the marriage of theoretical prowess and computational finesse that drives the wheels of progress, shaping the future landscape of physical chemistry.
The beauty of molecular modeling lies in its ability to bridge the chasm between theory and practical applications. It provides a platform where theoretical conjectures meet real-world validations, where hypotheses evolve into validated scientific principles. This synergy empowers scientists to envision and design novel molecules or materials with tailored properties, expediting the process of discovery and innovation.
Embarking on the journey through molecular simulations is akin to stepping into a multidimensional universe. It's a world where molecules become the protagonists, and theoretical concepts manifest into visualizations that transcend mere imagination. It's the thrill of unveiling the mysteries of molecular structures, deciphering their language, and predicting their behaviors with astonishing accuracy.
In this realm, the allure of physical chemistry magnifies. The magic of theoretical concepts comes to life through simulations, amplifying our understanding and igniting a passion for exploration. It's a playground where curiosity meets technology, where the enigma of molecular structures transforms into comprehensible models, empowering scientists to chart new territories in the vast landscape of chemistry.
Therefore, I beckon all aspiring minds to immerse themselves in the captivating world of molecular simulations. Witness the magic unfold, as theoretical concepts unfurl their wings and soar into the realm of reality. Embrace the tools of computation, for they are the keys unlocking a universe of possibilities, perpetually reshaping the frontiers of scientific exploration in the fascinating domain of physical chemistry.